Introduction
Explore nanotechnology and semiconductors, where atoms and molecules offer endless technological possibilities. This study examines the revolutionary changes caused by nanometer-scale semiconductor features. New nanoscale properties are changing our understanding of electronics and opening new avenues in memory, processing, and material science.
The Future of Semiconductor Innovation and Nanotechnology
The feature sizes of modern semiconductors are measured in nanometers during manufacturing. Despite this, the conventional classification of semiconductors as nanotechnology is not applicable. When compared to a transistor at the 32nm node, one made using 0.5µm technology does not exhibit significantly different behavior. In order for a material to be considered nanotechnology in the modern sense, it has to possess properties that deviate from mere dimension scaling.
The facts about them are affected by physics rules since the feature size is about the same as the critical size for physical events. The difference frequently shows up as a step change. Tens of nanometers, for instance, are usually the radius at the tip of a crack. Smaller than this, the material’s dimensions prevent conventional crack propagation and the ensuing mechanical failure.
There are two ways that nanotechnology might show up in the semiconductor business. The semiconductor devices themselves are the first of these. It is common knowledge that we cannot continue to use smaller devices forever. Quantum physics becomes relevant when a device’s size approaches that of a single atom; a transistor may or may not switch, depending on the current statistics. While it is not logical to construct conventional logic gates from these kinds of devices, alternative quantum-based decision-making architectures are in the process of development.
A crucial parameter of the substance utilized in solid-state memories is the change ratio between the 1 and 0 states. A well-made and engineered flash memory will have a ratio of about 10,000. It is possible to achieve conductivity ratios greater than one million by taking advantage of the phase change in the nanomaterial graphene. This would yield five times more storage capacity if implemented in a full-sized memory.
The Quantum Size Effect’s (QSE) Benefit
When small crystalline structures, like semiconductor materials, shrink more rapidly, their electron and hole quantum confinement increases. This phenomenon is known as the quantum size effect (QSE). A molecule’s molecular size falls between that of its bulk material and that of an affected nanomaterial due to changes in the electronic structures of these nanomaterials caused by QSE. When this transitional state is present, a special change occurs in the DOS of the valence and conduction bands of semiconductors and metals, causing charge carriers to be spatially enclosed within these structures.
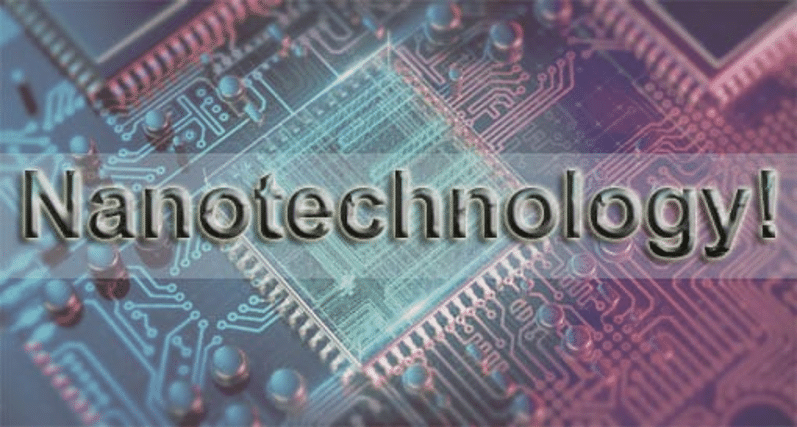
Manufactured nanomaterials can display striking changes in their physical properties from bulk materials due to the electron structural alterations resulting from QSE. Researchers can alter the energy and optical transitions of semiconductor materials that have been nanoengineered by varying the dimensions and configuration of these intermediary atoms. Depending on the intended use of the material, researchers can, for instance, change the electronic energy state of the nanoparticles to modify the light emission that can pass through them to be in the ultraviolet, visible, near-infrared, and mid-infrared spectral ranges.
Using Semiconductor Nanomaterials in Applications
When contrasted with their more traditional bulk and molecular counterparts, semiconductor nanomaterials exhibit intriguing physical and chemical characteristics as well as practical functions. The most desirable characteristics of these materials are their processability, surface functionality, strong chemical and photobleaching stability, continuous absorption bands, narrow and intense emission spectra, and surface functionality. Countless articles detailing methods for creating semiconductor nanoparticles attest to the growth of “nanochemistry.”
Consider the substantial alteration in semiconductor nanomaterials’ optical properties caused by the spatial quantum confinement effect. Extremely high dispersity, or a high surface-to-volume ratio, combined with the semiconductor’s chemical and physical characteristics, greatly affect its surface and optical characteristics. Consequently, for the past 20 years or so, researchers have concentrated on semiconductor nanomaterials. They have generated a great deal of interest in a wide range of fields, including materials science, inorganic chemistry, solid-state physics, physical chemistry, colloid chemistry, and, more recently, biological sciences, engineering, medical sciences, and interdisciplinary fields.
Conclusion
The semiconductor industry is entering a new era thanks to nanotechnology. Scientists find this small world of unexpected material behavior fascinating, and it holds great promise for future technological advancement. Material science and electronics are entering a new era with quantum effects changing material makeup and semiconductor nanomaterials transforming industries. These advances promise a time when nanoworld wonders will push technology to new limits, redefining what is possible.